Young at Heart: Recent Advances in the Genetics of Congenital Heart Disease and Translational Care-Juniper Publishers
Abstract
Congenital Heart disease affects almost 1% of babies born in the UK, but few receive a genetic diagnosis. Identifying a genetic cause is important for that individual’s healthcare, and that of their family. It enables accurate genetic counselling, including discussion of reproductive choices. Significant advances have been made in identifying genes that are involved in the developing heart and congenital heart disease, and research has moved away from single family studies towards genetic sequencing of large cohorts. This will bring new challenges for clinicians and researchers related to data sharing and incidental findings. In the future, we hope that new treatments can be developed based on our understanding of the complex genetic networks underlying development of the heart.
Keywords: Congenital heart disease; Genetics; Inheritance; Chromatin; Sequencing; Syndromic; Non-syndromic
Introduction
Congenital Heart Disease (CHD) encompasses a wide range of defects and affects 7-9 per 1000 births in the UK [1,2]. It has a higher mortality than many other birth defects [1,3] and there is a significant genetic component to CHD. However, straight-forward mendelian inheritance is uncommon, and in the majority of cases there is a complex pattern of inheritance or a single affected family member. The developing heart relies on a specific pattern of gene expression and mutations in a number of these genes have been identified to cause CHD already. Reduced penetrance and variable expressivity suggests that there are additional factors or other genes involved too. The chance of recurrence varies between the different defects, and certain types of CHD seem to occur together more often than others [4]. Family screening has been advised for defects such as bicuspid aortic valve and hypoplastic left heart, but may not be appropriate in all cases, given the heterogeneity of the structural lesion and inheritance patterns [5]. Individuals with CHD are now living longer due to better medical and surgical care, with 95% reaching adulthood, and most have an active life [6]. As a result, they now represent a considerable cohort of individuals requiring specialist medical care. In addition, they are more likely to survive to reproductive age and may ask about the risks of their children being affected.
Aetiology
CHD is thought to arise due to a combination of genetic and environmental factors. Contributing factors are summarised in Figure 1 [7-10] and include a number of maternal factors. Currently a genetic diagnosis can be identified in 10-20% of individuals with CHD. The history should include a three generation family tree, including neonatal demise. Additionally, exposure to teratogens, alcohol and illicit drug use may be relevant. A more generalised examination may provide useful clues to a specific diagnosis, such as limb defects or dysmorphic facies. The chance of making a diagnosis is increased if an individual has syndromic CHD (S-CHD), compared with non-syndromic CHD (NS-CHD). NSCHD is CHD in isolation, S-CHD is the combination of CHD and another congenital abnormality or neurodevelopmental disability, or both. Syndromic CHD (S-CHD) only makes up around 10% of all CHD, so the vast majority of people with CHD unfortunately do not receive a genetic diagnosis.

Genetic causes of CHD include chromosome abnormalities, copy number variation and single gene disorders. Around 10% of CHD is thought to result from chromosomal abnormalities, which can be detected using karyotyping and array-CGH [11]. Trisomy 13, 18 and 21 commonly result in CHD. Turner Syndrome (45X) is another example. Smaller structural abnormalities such as 22q11.1 deletion syndrome and Williams Syndrome (deletion of 7q11.23) are also associated with CHD. Other examples include Wolf-Hirshorn (4p-), Cri-du-chat (5p-) and deletion of 1p36. Copy number variation (CNV) also contributes to CHD. CNVs are smaller deletions and duplications that can be detected by array-CGH, but would not be evident on a karyotype. There are now a number of recognised microdeletion and microduplication syndromes that include CHD as part of the phenotype, such as the 1q21.1 microdeletion [12]. Reduced penetrance appears to be relatively common amongst the microdeletion and duplication syndromes, which makes genetic counselling much more challenging.
Around 5% of CHD is thought to result from single gene disorders [13]. Mutations in a number of genes causing CHD have been described and include those encoding transcription factors and cardiac structural proteins (Table 1). Epigenetic factors such as chromatin and histone remodelling, and DNA methylation are important in controlling which genes are expressed. A number of genes involved in chromatin regulation are already linked with CHD such as KMT2D, KDM6A (Kabuki Syndrome) and CHD7 (Charge Syndrome). Somatic mutations in the heart are only thought to play a small role in the pathogenesis of CHD [14].
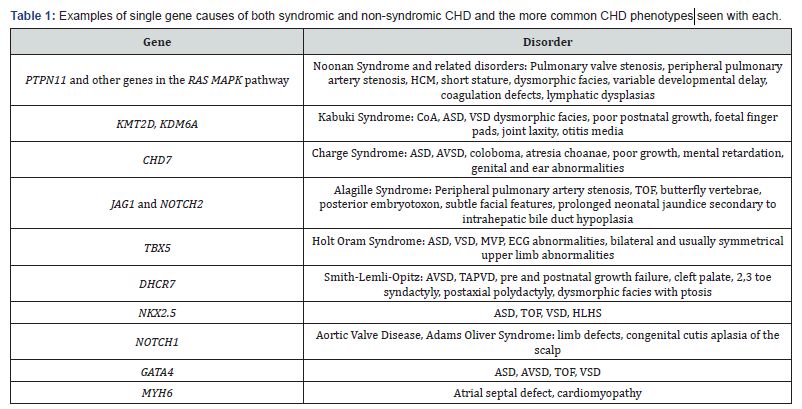
Identifying novel CHD genes
Traditionally novel pathogenic genes were identified by studying families with multiple affected members. Subsequently, targeted sequencing of a candidate gene might be carried out in a larger cohort of individuals with the same phenotype. Both of these strategies may fail to uncover the true phenotypic spectrum associated with a particular gene and there is always a risk of missing another pathogenic mutation. It also does not provide an accurate incidence of CHD due to that specific gene and functional follow up studies are not always carried out. More recently, whole exome sequencing (WES) has been used in large cohorts of individuals with CHD to identify novel genes that cause CHD. The number of mutations identified in the CHD cohort can be compared to the number expected by chance and control populations. Mutations can be stratified by type and their predicted effect on the gene, including loss of function, gain of function, protein truncating, missense and synonymous. Analysis of the proband and parent’s DNA allows us to determine if the mutation is de novo or inherited.
Studies have shown that individuals with CHD have more de novo loss of function and predicted deleterious missense mutations than expected by chance and compared to controls [15-17]. In addition, they show an increased number of mutations in genes that are highly expressed in the embryonic mouse heart which are likely to be important in the formation of the heart. De novo protein damaging mutations in these genes are thought to account for 20% of individuals with S-CHD and 2% of isolated CHD [16]. Genes involved in histone and chromatin modification appear to be especially important [17,18]. Novel CHD genes uncovered using this approach include CDK13, CHD4, PRKD1, RBFOX2 and FLT4. Recessive causes identified include a GDF1 founder mutation, and recessive genotypes have been seen in genes previously associated with dominant disease (MYH6) [19]. These results suggest that genetic aberrations play an important role in the pathogenesis of CHD.
These WES based studies have also provided an insight into the inheritance of CHD (Figure 2). S-CHD was postulated to be a
result of de novo mutations, and this was confirmed by Homsy et al. [16]. However, for NS-CHD a significant excess of mutations that truncate the protein, was identified in genes inherited from healthy parents [15]. This suggests these mutations exhibit reduced penetrance and goes some way to explain the apparently sporadic nature of NS-CHD in many families.Translational aspects
Since the initial description of individuals with CHD and mutations in CDK13 and CHD4, further case reports have been published [20,21]. The phenotype of these specific syndromes will likely continue to expand and a distinct facial gestalt for CDK13 and CHD4 has been recognised. This will hopefully mean that clinicians are able to identify affected individuals and instigate genetic testing. Describing the non-cardiac phenotypes will also guide further investigations and give information on prognosis. Both of these genes show reduced penetrance for CHD, 59% of individuals with CDK13 mutations have CHD and 70% of those with CHD4 mutations. The mechanism behind this is still unclear but not unexpected given that reduced penetrance is a common theme in CHD.
Making a genetic diagnosis may not alter the medical treatment offered to an individual, but it can still provide important information. Making a syndromic diagnosis may mean that additional screening of other organ systems is offered, as in Noonan Syndrome for example. The individual may wish to consider pre-conceptual counselling, genetic testing in a pregnancy, or even preimplantation genetic diagnosis. Cardiac screening can be offered to other family members in some instances, but genetic testing may offer a more definitive answer and mean that only those who are mutation positive require further investigations. In the future, we may be able to identify a genetic cause of CHD in more people and provide more tailored medical care [7-10]. Understanding the network of genes involved in CHD may open up different treatment avenues for investigation, such as the use of stem cells.
Conclusion
Although significant progress has been made in understanding the genetic contribution to CHD, there is still some way to go. A number of novel genes have been described and the different contribution of de novo and inherited variants to S-CHD and NSCHD has been identified. What has become apparent is that we will need to analyse far bigger cohorts, ideally DNA from tens of thousands of individuals [15]. Such a vast study will require collaboration between many research groups and clinicians. This brings with it the challenges of big data analysis, data sharing and confidentiality of genetic data. Research teams and clinicians should consider the possibility of incidental findings, such as a mutation in a tumour suppressor gene or carrier gene status when sequencing exomes or the whole genome. In addition to the genetic contribution, the importance of environmental and parental factors should not be neglected. Factors such as maternal obesity may become more relevant given the increasing rates of obesity worldwide. Health services should also adapt to care for the increasing numbers of adults with CHD and the specific needs they may have, including genetic counselling. Hopefully in the future, new therapies can be developed for both CHD and the failing heart based on our increasing knowledge of the genetic controls of heart development and their interaction with the environment.
Acknowledgement
A Wilsdon is a British Heart Foundation Sponsored Clinical Research Fellow FS/14/51/30879.
For more Open Access Journals in Juniper Publishers
please click on: https://juniperpublishers.com/open-access.php
For more articles in Open Access Journal of Cardiology & Cardiovascular Therapy please click on: https://juniperpublishers.com/jocct/index.php
Comments
Post a Comment